Food for Thought
When Ewan McNay was eight years old, he decided that he wanted to do two things: live forever and find out how the brain works. The secret to eternal life is a work in progress, but his research on insulin resistance in the brain has added a new dimension to our understanding of Alzheimer’s disease.
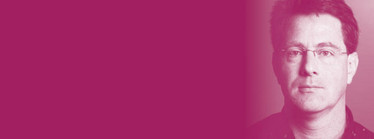
Alzheimer’s disease is a story that goes from a single atom all the way up to a human and even societal scale. My work focuses on the link between Alzheimer’s and insulin-resistant diabetes, which first came to light in the late 80s and has progressed over the past 20 years to a point where we have a good understanding of at least some of the molecular bases linking the two diseases, and can even take an educated guess on how we might be able to intervene. People with Type 2 diabetes are up to seven times more likely to develop Alzheimer’s disease, but you don’t have to be diabetic for insulin resistance to affect the brain. It’s perfectly possible to have impaired brain insulin signaling, even if your body is not systemically insulin resistant – an observation confirmed in post mortem studies of Alzheimer’s affected brains. We suspect that, in some patients, brain insulin resistance is a consequence of a whole-body disease, while other patients have what is effectively a brain-limited form of diabetes – sometimes referred to as “Type 3 diabetes”.
Both beta amyloid and insulin are broken down by insulin degrading enzyme (IDE). In Type 2 diabetes, the body fails to respond to insulin, so the body produces more in an attempt to overcome this lack of response. IDE preferentially breaks down insulin over beta amyloid so beta amyloid builds up in the brain while IDE is occupied breaking down insulin. That accumulating beta amyloid causes several problems, one of which is to bind to insulin receptors and further dampen insulin signaling – a downward spiral. Specifically, beta amyloid impairs a glucose transporter called GluT4, which removes glucose from the blood in response to insulin. In the brain, GluT4 is found in a very specific subset of brain pathways – one of which is the hippocampus, key to memory – which matches up with where you see the deficits in Alzheimer’s and some other dementias. The ability of the hippocampus to respond to intellectual challenges by increasing glucose metabolism is impaired or absent in the Alzheimer's brain, causing cognitive impairment in these patients.
We were the first to discover both that insulin acts to regulate hippocampal metabolism and memory, and that if you block endogenous insulin in the rat hippocampus, it causes a big memory lapse. We then showed that if you introduce excess beta amyloid, you see similar impaired insulin signaling, impaired metabolism, and impairment in memory. We predicted that if we examined the brains of rats with the equivalent of Type 2 diabetes, we would see elevated levels of beta amyloid, impaired brain translocation of GluT4 and reduced glucose metabolism. And sure enough, we saw all three.
Next, we asked whether blocking beta amyloid in the hippocampus could reduce cognitive impairments. In our most recent work, we showed that it did, at least in animal models. We used a rat model of Type 2 diabetes, feeding them a high-calorie, high-fat diet to induce obesity and insulin resistance: we had previously shown that this treatment also causes hippocampal accumulation of beta amyloid. As expected, the rats began to develop cognitive impairment. Working with a protein engineer at Rensselaer Polytechnic Institute we produced antibody fragments targeted against particular forms of beta amyloid. Delivered directly into the hippocampus, the antibody fragments mop up oligomeric beta amyloid – the toxic form that eventually forms plaques – and, as predicted, cognitive impairment was reversed (1).
Unfortunately, we are currently administering these antibody fragments directly into the brain – not a plausible intervention route for humans. It’s very unlikely that we could give that antibody fragment orally or even intranasally. If someone can crack the challenge of how to get the appropriate drug treatments to the brain then that conversation can start to take place. Right now, there is no way for us to do that, but our studies do suggest that there are lifestyle choices we can make that are likely to reduce our risk of dementia in later life. Your brain is not floating in a vat: it is part of the biological system of your whole body. Habits that affect the rest of your body can and do have long-term consequence in your brain. At the moment, the evidence suggests that you are less likely to get Alzheimer’s if you:
- Maintain a healthy weight to prevent Type 2 diabetes
- Exercise regularly
- Drink coffee and red wine (in moderation) – both are linked to reduced risk of dementia, possibly because the antioxidants they contain protect the brain from damage
Right now, we’re working on confirming our hypothesis that GluT4 is a point of common action by which insulin boosts, and beta amyloid impairs, brain metabolism and memory. If it is, there could potentially be therapeutic benefit in upregulating GluT4 to allow more fuel to reach your brain cells. Tight regulation of brain metabolism is essential – if you just put the brain into overdrive, you might get a temporary increase in function but end up causing oxidative damage to the cells – imagine permanently running your car at 7000 rpm... A better understanding of the molecular-level interactions will tell us whether there might be some way to improve function without “blowing the engine”.
Ewan McNay is Associate Professor of Behavioral Neuroscience at the University at Albany (SUNY), USA.
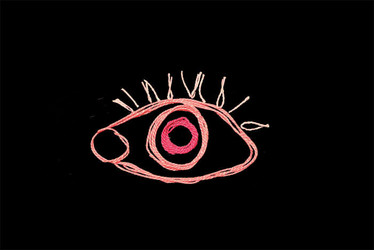
One to watch
Shraddha Sapkota, University of Alberta, caused a stir at the 2015 Alzheimer’s Association International Conference with her work on analyzing saliva biomarkers to detect Alzheimer’s disease. We caught up with her to find out more.
Could you briefly describe your work?
We used cutting-edge liquid chromatography–mass spectrometry at the University of Alberta to analyze saliva samples from patients with normal cognitive function, with mild cognitive impairment or with Alzheimer’s disease. We were looking for perturbations in metabolites that represent early biological markers of disease processes. Metabolomic analyses allow us to sort through thousands of metabolites to determine the top biomarkers that discriminate different clinical groups. In this case, we were able to clearly discriminate patients with Alzheimer’s disease from those experiencing mild cognitive impairment and the other comparable non-impaired older adults. Analyses also provided information about the possible biological pathways from normal aging to Alzheimer’s disease. Finally, we used the detected biomarkers to predict preclinical manifestations of the disease – specifically, early cognitive decline.
What’s next?
We are working towards the identification of key biomarkers that reflect the earliest changes indicative of preclinical Alzheimer’s disease and possible mechanisms. These changes can begin to appear more than 10 years prior to clinical diagnosis, so we are aiming for tests that can be used in middle-aged adults, especially those experiencing some memory impairment. However, we are still at the early stages and much work is needed before we can include saliva tests for the general population.
Could it help advance research?
We have received a lot of interest from the research community. Aside from diagnosis, salivary metabolomic analyses could promote our understanding of the mechanisms from normal aging to Alzheimer's disease – and provide objective biological endpoints to compare different risk factors and interventions. Saliva is very easy to collect and transport, which could enhance participation in remote centers and diverse populations.
Untangling Tau by Brad Hyman
Iron’s Influence by James Connor
The Overactive Brain by Michela Gallagher
- E. McNay, D. Osborne and P. Tessier, “Single-domain antibodies that bind oligomeric beta-amyloid reverse cognitive deficits produced by high-fat diet-induced obesity”, Society for Neuroscience Annual Meeting, San Diego, USA (2013).
Ewan McNay is Associate Professor of Behavioral Neuroscience at the University at Albany (SUNY), USA.