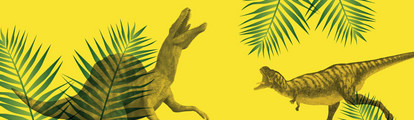
CSI: Cretaceous
The people who investigate disease and death in dinosaurs
At a Glance
- “Molecular paleontology” is the study of ancient biomolecules preserved in fossilized bone
- Practitioners of the unusual discipline use techniques familiar to modern pathologists and laboratory medicine professionals, but face additional challenges
- Certain conditions, such as terrestrial environments and rapid burial, improve the chances of soft tissue preservation
- Interdisciplinary research involving both modern medicine and paleobiology can yield great strides in molecular paleontology studies
What killed the dinosaurs? You may immediately think of an asteroid impact, a dramatic climate shift, or possibly an alien invasion – after all, the cause of their mass extinction is still somewhat open to scientific debate (although aliens have largely been ruled out). But what about the dinosaurs who died before the Cretaceous-Paleogene extinction event? Were all of their deaths dramatic predator-prey interactions? Did they suffer from cancer, arthritis, or even plain old age?
We’ve previously explored the unique careers of those who study the pathology of past peoples, whose patients may be hundreds or even thousands of years old (1)(2). But just as not all modern pathologists treat human patients, not all paleopathologists investigate the history of human disease. Some study much older – and, in most cases, much larger – patients: the “terrible lizards.”
But believe it or not, even the paleontologists and pathologists who study dinosaurs over 100 million years old use many of the same techniques on which modern laboratory medicine professionals rely. “Molecular paleontology” labs feature light and electron microscopes, mass spectrometers, immunohistochemistry platforms, synthetic peptides, and more. Practitioners of this discipline combine a paleontologist’s work in identifying and extracting fossil material with the laboratory medicine skills of analyzing the ancient biomolecules preserved in fossilized bone. It’s a science that works across interdisciplinary boundaries to yield great strides not only in paleontological studies, but also in the practice of modern molecular biology.
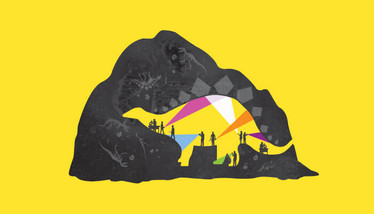
Pathology Meets the King of the Dinosaurs
What’s the most unusual patient you’ve ever encountered? Many pathologists and laboratory medicine professionals will recall unusual tumors, rare disorders, or even – for some – a unique veterinary specimen. But how many can claim that their standout examination has been conducted on a dinosaur? And not just any dinosaur, but a true Cretaceous celebrity: a Tyrannosaurus rex?
That’s precisely the patient Mary Higby Schweitzer dealt with in 1993, when she noticed odd structures within fossilized bone. Faced with the possibility that those structures might be red blood cells – a tissue whose survival from ancient times was previously unanticipated – she delved deeper into the search for soft tissues preserved for millions of years. Ultimately, she was able to identify and isolate collagen, possible red blood cells, protein sequences, and even preliminary evidence of DNA from dinosaur fossils, revealing fascinating new information about how biomolecules can be preserved and how they compare to modern examples.
Fortunately, a Jurassic Park debacle is unlikely – but modern pathology and laboratory medicine still have much to gain from the study of these ancient tissues. We spoke to Mary Schweitzer to learn more…
You practice “molecular paleontology” – an unusual term…
Paleontology is the study of “old life.” Vertebrate paleontology is the study of old bones, and molecular paleontology is the study of old molecules. It’s the study of biological molecules recovered from ancient bone and the methods the discipline’s practitioners freely borrow to determine those molecules’ endogeneity. We apply well-tested methods to recover these tissues and molecules and then analyze them using many of the same techniques modern pathologists, osteologists, and protein chemists use. It is certainly a far less straightforward discipline, though, because we have to account for chemical changes that have accrued on the molecules within fossilized bone – ones that happy, healthy, modern molecules don’t contain. And that means developing new (or modified) protocols for our analyses. We also have to account for the fact that comparative databases consist mostly of mammals, which somewhat limits what we can do with dinosaur tissues.
How did you become a molecular paleontologist?
I never thought I had what it took to be a scientist. I am pretty badly handicapped in mathematics, and chemistry and physics are very much like math. In addition to fearing science, I was also kind of lazy! My first degree was in “educational audiology,” which is basically deaf education with a clinical component. I loved it, but I wasn’t ready to get the advanced degree I would have needed to practice it. Instead, I got married and had kids – and I decided that, when my kids went off to school, I would get a teaching certificate so I could be where they were in high school. (One of their greatest fears, as I’m sure you can imagine!)
As I was finishing the certificate, I noticed that famous paleontologist Jack Horner was teaching a class. It was mid-year and I couldn’t get a job, so I sat in on the class. I guess you could say that my curiosity overcame my fear of science. I continued as a volunteer for a while, but I had a million questions and, finally, Jack ran out of answers, so he told me to go and get my own degree.
I signed on for a Master’s program, proposed my thesis ideas to my committee and, the day after my committee met, a bone expert sent me back a microscope slide of a T. rex bone I was working on. She said to me, “Did you know there are red blood cells in this bone?” But you cannot determine this from morphology alone. After 65 million years in the ground, virtually any chemical reaction is possible. So I proposed some alternatives – for instance, sedimentary structures or pollen – and tried to eliminate those options with data. The rest is history.
No one thought soft tissues could be discovered in fossils – so how did you do it?
It started with the sighting of those little round structures inside the vessel channels in the T. rex bone. Jack said, “What do you think they are?” I said that I knew it wasn’t possible, but they were the right size, shape, and location for red blood cells. They were even iron-rich and nucleated, so they fit every criterion. His response was, “So prove to me that they aren’t.” That formed the basis of my dissertation – the attempt to prove that these weren’t red blood cells. In the end, of course, I couldn’t disprove it, but I did accumulate a lot of chemical (despite hating the subject!) and other evidence for the preservation of molecular components.
When I took a job at North Carolina State University, I wanted to repeat those studies to see if I could figure out what was going on. Jack sent me a box of fragments from a new T. rex discovery, that had not been treated with any chemicals; they were “fresh out of the ground.” I pulled the first big chunk out of the box, turned to my technician, and said, “Oh, my gosh! It’s a girl, and she’s pregnant!” I believed I was holding medullary bone – the estrogen-dependent reproductive tissue birds produce during lay.
In an effort to determine whether or not this new tissue I saw really was what I thought, I went to the literature to see how bird medullary bone was studied and found that they always removed the mineral phase of the bone to study the organization of collagen in medullary bone, which is different to that of other bone types. We didn’t think that would work in a fossil of this age, because conventional wisdom states that organics don’t preserve – so if we removed the mineral phase, we assumed there would be nothing left.
I told my tech to do just a short etch to reveal the pattern of the collagen fibers that had once been there. The process went on longer than I had intended… Imagine our surprise when, not only did the bone not completely dissolve (as it should have if we had been right about the organics’ degradation), but it actually left a stretchy, fibrous matrix like modern bone! We repeated the process because I thought it had to be wrong – a fluke – but no, it was very consistent. Then, I tried it on regular cortical bone, not the “pregnant” tissue. That’s when we observed the vessels and cells. But we had to remember an important lesson in paleopathology: just because it looks like something, after 70 million years, that doesn’t mean it is. With that in mind, we turned to chemical and molecular methods to satisfy our curiosity (did I mention I hate chemistry?).
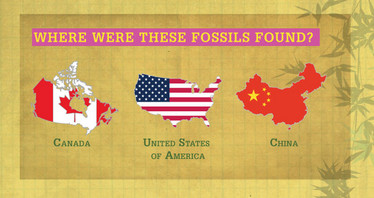
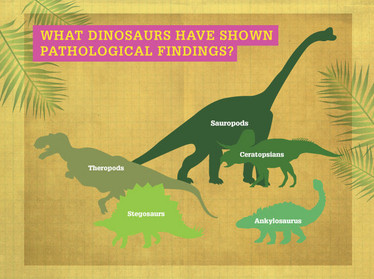
What features make a fossil a good potential source of soft tissue?
After our initial findings, we applied our methods to a time point study, looking at bony remains from fossils that spanned multiple ages, taxa, depositional environments, and continents. We looked for the presence of structures morphologically consistent with collagen, blood vessels, and cells – and we found soft tissues were present in one-half to one-third of all fossils we examined. It does seem, though, that it was better to be buried in sandstone than in mudstone, in terrestrial versus marine environments, and to be buried rapidly and deeply. So those were our starting points when looking for a new dinosaur.
Since that time, I have become convinced that the full answer is a lot more complicated – and, for the time being, I don’t know everything involved. I think that, to start, you should have a fossil that demonstrates unusual preservation – articulated, preserved with skin, or preserved in an environment that yields other pristine fossils. Then, you just have to look…
But how have these soft tissues remained intact for so long within fossils when they degrade so quickly under laboratory conditions? I think you have to account for the unique environment that exists in vertebrate bone. Bone mineral is microcrystalline, with a huge surface area and a strong affinity for organics. In fact, researchers have even purified DNA on apatite columns. Additionally, the way bone is structured makes it difficult for microbes to access the internal-most parts. I also think iron, as a reactive oxygen species, has a lot to do with preservation (3)(4), and iron is released into the local environment in large quantities when a T. rex drumstick degrades.
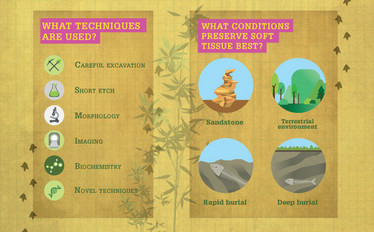
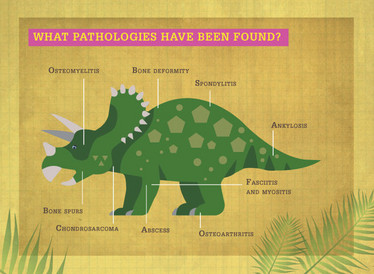
How did you handle pushback from researchers who doubted your conclusions?
It was (and is) really hard sometimes. With everything we publish, we try to set rigorous standards and never overstate our data. We never publish unless we can repeat our results three times, and we never ever publish using just one or two methods. In fact, I even had a reviewer recommend rejecting one of my papers because there was “too much data!” But there are some who will not be convinced with data – and, rather than accept the evidence we provide, say they don’t “believe” our data or challenge our methods. Antibodies, for instance, can cross-react with structures containing epitopes of a similar shape to their target proteins. As a result, I’ve been told that they can yield “false positives,” which is not quite the case, and which risks their being devalued as a molecular paleontology technique. Rather, these types of inaccuracies are the reason we use controls!
I was taught that, if I wanted to challenge work in the peer-reviewed literature, I had to come up with an alternative explanation that cohesively fit all of the published data and explained the conclusions equally well, and then come up with additional data that better supported our alternative than the original hypothesis. Generally speaking, our challengers have not applied this standard. Typically, they fail to acknowledge many of the lines of evidence we produce, addressing only the one that they try to disprove. Or, when they purport to “test” our hypotheses, they use methods different to those that we used. I don’t think that is a valid trial.
So how do I handle it? I yell and stomp around and whine to my friends and get really snarky for a day or two – and then I try to see the value in the criticism. Ultimately, I go out and try to produce more data. Even the very worst reviews contain something I can learn from.
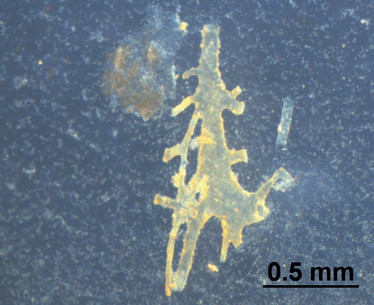
A fragment of a fossilized T. rex blood vessel.
You’ve found evidence of DNA in dinosaur fossils. What could we potentially learn from that?
Evidence of the possible presence of DNA in fossilized bone is, to me, just one more piece of evidence that proteins could persist – rather than having proteins be a step toward the eventual goal of DNA recovery. I’m told that this is a rather unusual attitude! DNA is not the focus of our research, nor is it the end goal. DNA is not what natural selection operates upon; rather, it is the expression of DNA as proteins. Now, of course, if we have DNA we can predict protein sequences and go looking for them. However, the DNA sequences don’t say much about what the end product – the functioning, three-dimensional protein – may look like. And that folded, convoluted, final protein is what determines its function.
It is predicted that DNA has a shorter half-life than proteins. I don’t put much weight on such predictions, but if I am really interested in non-avian dinosaurs, it doesn’t make a lot of sense to start with DNA because of its predicted lack of longevity. However, if DNA is there, then proteins surely should be – just as, if proteins are there, we should be able to see the persistence of lipid-based compounds, which are predicted to last longer still. In fact, we recently published evidence of blubber in an ichthyosaur fossil (5), based on its resemblance to other lipid-rich fossils and the presence of potential fatty acid-derived moieties.
What techniques do modern paleontology and laboratory medicine share?
As far back as the 1950s, paleontology started to borrow from modern osteology by investigating fossil bone at the microscopic level. This was pioneered by Donald Enlow and colleagues, but not really followed up on until the 1980s, when Armand de Ricqlès, Kevin Padian, and Jack Horner brought it to the forefront. Now, most paleontological studies involve histology in one form or another.
Molecular analyses are off to a rather slow start. I think, though, that the broader community is beginning to see the power of molecular methods and the added information they provide, not only for elucidating phylogenetic relationships, but also for demonstrating function and evolutionary mechanisms. In the future, we hope to make de novo sequencing more efficient for what we do. One of our big problems, as I said above, is that most of the databases out there for molecular studies are mammalian – and mammals are not a great comparator when searching dinosaur sequences.
I believe strongly in the power of interdisciplinary research – including collaborations between paleobiologists and laboratory medicine professionals. There is so much to learn that no one person can learn it all. I am deeply honored to work with the colleagues I have across the board, and very humbled that they, for a moment, put aside some of their own projects to follow up on this crazy idea that molecules don’t necessarily always have a time limit.
My goal for work produced in my lab is that it be as robust and well-documented as possible. The reason I have never published a paper using just one method (nor will I ever) is that all methods are limited. The multiple methods we use are a great cross-check; they test different aspects of a molecule and validate its presence in ancient tissues. Each method we use contributes another piece of information about the functioning molecule, and thus the functioning organism. Large interdisciplinary studies require a lot of trust, though, so it’s good to be familiar with the people you work with and their reputations. I have worked with some of the best people out there. They think of stuff I haven’t; they employ methods I can’t; and I have been deeply honored to work with great teams.
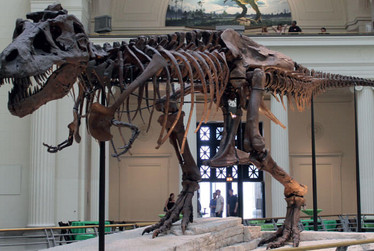
Sue, the famous T. rex, exhibits signs of possible gout, avulsion injury, and five other pathologies. Credit: Connie Ma
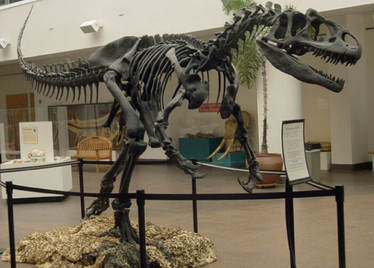
Allosaurus fragilis fossils show multiple bone pathologies, including the highest number of stress fractures in any theropod.
Do you have any advice for laboratory medicine professionals interested in this kind of work?
I think one of the huge potentials of the work we are trying to do is its applicability to medicine. Co-evolution of diseases/pathogens and hosts is a huge new area of study, but most are based upon the distribution of disease vectors among living hosts. They don’t really address (except by inference) questions like when and how these diseases started, when they invaded new lineages, or how they have been modified to become less virulent. Perhaps a disease plaguing us now had its start in ancient hosts. Can we probe those skeletal elements to identify them?
In addition, by studying how modern molecules are changed from ancient ones, we may gain insight into the progression of modern disease. We have found that iron-mediated crosslinks are key to the preservation of these tissues and molecules, but of course they are pathological – or lethal – to living organisms. But we know the molecular makeup of happy, healthy blood vessels, and now we have an end product. Can we find a pathway? And, if so, can we perhaps find a way to interfere with the process?
Of course, if you want to pursue this line of inquiry, you cannot do it in a lab where extant animals are routinely examined. There is just too much potential for crossover. Our labs (extant and ancient samples) are completely separate, and we never exchange anything between labs. This is predictably expensive, and it’s probably a major contributing factor in why these types of studies are not yet common.
For those who are interested in pursuing a similar line of study, I want to emphasize that it is very, very expensive and very high-risk in terms of return on investment. And yet, if we are not willing to make that investment, it becomes a self-fulfilling prophecy that molecules are not useful past a certain age limit. Our work is time-consuming and repetitive, so one project requires more than one person to do it right.
When we apply to funding agencies, we are always referred to geology/earth science-based programs, but we require a molecular biology budget. I think that we must rely more and more on private funds to do this kind of work. I particularly struggle with the ethics of recruiting students, although I love to work with them – I truly believe that they are the future. But if I still have so much trouble with funding after 25 years, can they make a career doing this? Perhaps therein lies the benefit of interdisciplinary research. If molecular paleontology and modern laboratory medicine team up, who knows what we could learn?
Mary Schweitzer is Professor of Biological Sciences at North Carolina State University, Research Curator at the North Carolina Museum of Natural Sciences, Research Associate of Paleontology at Montana State University’s Museum of the Rockies, and a Visiting Professor of Geology at Lund University, Sweden.
- N Lynnerup, “Connecting Past to Present”, The Pathologist, 47, 20–26 (2018). Available at: bit.ly/2V8Q34T.
- J Buikstra, “Bioarcheology: Peopling the Past”, The Pathologist, 47, 27–29 (2018). Available at: bit.ly/2JTVnaR.
- MH Schweitzer et al., “Molecular analyses of dinosaur osteocytes support the presence of endogenous molecules”, Bone, 52, 414–423 (2013). PMID: 23085295.
- MH Schweitzer et al., “A role for iron and oxygen chemistry in preserving soft tissues, cells and molecules from deep time”, Proc Biol Sci, 281, 20132741 (2013). PMID: 24285202.
- J Lindgren et al., “Soft-tissue evidence for homeothermy and crypsis in a Jurassic ichthyosaur”, Nature, 564, 359–365 (2018). PMID: 30518862.

While obtaining degrees in biology from the University of Alberta and biochemistry from Penn State College of Medicine, I worked as a freelance science and medical writer. I was able to hone my skills in research, presentation and scientific writing by assembling grants and journal articles, speaking at international conferences, and consulting on topics ranging from medical education to comic book science. As much as I’ve enjoyed designing new bacteria and plausible superheroes, though, I’m more pleased than ever to be at Texere, using my writing and editing skills to create great content for a professional audience.