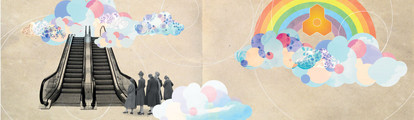
Positive Steps to Tackle Triple-Negative Breast Cancer
How to distinguish between different molecular subtypes of TNBC – and why it’s so important
At a Glance
- Triple-negative breast cancer (TNBC) is not a single entity, but rather multiple distinct molecular subtypes
- Each TNBC subtype shows different alterations in gene expression and has the potential to be targeted by different types of therapy
- To develop better TNBC therapies, we need to establish exactly what constitutes “triple-negative” and find a cost-effective way of identifying such tumors
- Digital image capture, artificial intelligence, and deep learning may help us spot patients who would benefit from TNBC targeted treatment
No two breast cancers are the same – and the more we learn about each type, the more we uncover the truth of that statement. Once classified only by location, then later by hormone receptor status, we are now discovering new molecular subtypes of each breast tumor. This includes the triple-negative breast cancers (TNBCs), noteworthy for their lack of expression of nuclear hormone receptors and HER2. These tumors typically have poor outcomes and cannot be treated with hormone therapies like their positive counterparts; however, the discovery of multiple distinct molecular subtypes of TNBC means that detailed analysis could lead to better targeted treatment and, ultimately, improved outcomes for patients.
Current testing in breast cancer
At the moment, we diagnose breast cancer by pathological assessment of the diseased tissue. A histopathologist assesses the microscopic morphology of the tumor to confirm the clinical diagnosis of breast cancer and to assess features – such as type, grade, and lymphatic invasion – that are prognostic of cancer outcome. The pathologist then applies a set of predictive analyses to the tissue, using immunohistochemistry (IHC) to determine specific protein expression or in situ hybridization (ISH) to detect gene amplification. These techniques are all microscopy-based, meaning that a pathologist is needed to identify the correct cells for analysis.
The standard predictive tests include IHC detection of expression of the nuclear hormone receptors – estrogen receptor (ER; see Figure 1) and progesterone receptor (PR), which are predictive of response to hormone therapies – and either IHC or ISH detection of HER2 status (Figure 2) to determine which patients may benefit from HER2 targeted therapies. Expression of these three proteins is also prognostic of overall outcome. In addition, many laboratories perform IHC detection of the Ki-67 protein, a marker of cell proliferation that can be used both to determine the utility of chemotherapy and for an indication of overall prognosis.
The disadvantages of this current method of diagnosis is that the tests are semi-subjective. Why? Because they depend on the pathologist’s interpretation of the tissue. Of course, because pathologists undergo rigorous training, the subjectivity is kept to a minimum – but it still exists. Also, data quantification is difficult via histopathology techniques, which are essentially visual observations at a microscopic level.
Over the last decade, we have made great progress in understanding the molecular drivers of breast cancer – and, as a result, we understand much more about the complexities of how these cancers develop. Such analyses have also emphasized the different molecular types of breast cancer, underpinning the morphological diversity histopathologists have noted for many years. A major molecular dichotomy in breast cancer typing is whether or not ER is transcribed. There is then further stratification, at a gene transcription level, of ER-expressing and non-expressing cancers. To some extent, the lessons we have learned from the molecular analysis of breast cancers have led to a more formalized assessment of IHC prognostic and predictive analyses, with increased emphasis on PR expression as a surrogate for a functioning ER transcriptional activation pathway. (Why? Because a functioning ER pathway causes upregulation of several genes, including PR.) We also emphasize analysis of the proliferative activity of the tumor, beyond a mitotic count, using Ki-67 IHC.
Based on the data built up over the last decade via molecular profiling of breast cancer, there are now commercially available platforms for transcriptional analysis of diagnostic breast cancer samples, which can be used in addition to standard histopathology-based tests. At the moment, though, these panels are only discriminatory in the prognosis of ER-positive, HER2-negative, early-stage breast cancers. These tests are based on expression profiling of between 12 and 70 genes (depending on the platform) that aim to predict the long-term outcome of such a cancer and therefore to assess whether there is a need to use chemotherapy, as well as hormone therapy, in the management of a patient.
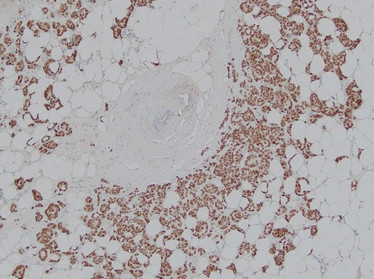
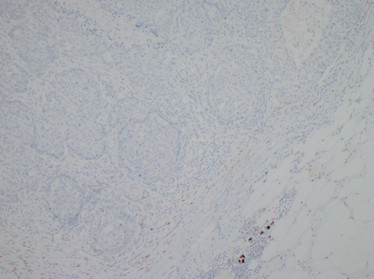
Figure 1. A) ER-positive breast cancer. IHC shows strong nuclear expression of ER in the breast cancer cells, which have infiltrated into fat. B) ER-negative breast cancer. The islands of breast cancer cells are seen across the top left. A few residual benign breast epithelial cells, which strongly express ER, are seen at bottom right. This indicates that the cancer is a true ER-negative breast cancer.
The nature of TNBC
Because it is simply a matter of detecting absence of expression of ER, PR, and HER2 in breast cancer cells, TNBC is not difficult to diagnose. However, as with any diagnosis based on absent expression, it is important to prevent false negatives. We can achieve this relatively easily with ER and PR, as long as we examine a slide that contains both breast cancer and normal tissue (because normal breast epithelium expresses ER and PR, which can be used as an internal positive control; see Figure 1b). Normal tissue can also be used as an internal control of HER2 expression – but HER2 overexpression should not be detected in the normal breast epithelium, so HER2-negative cancers will have similarly minimal expression, whereas a HER2-positive breast cancer will show strong overexpression (Figure 2a). Additionally, because HER2 protein overexpression in breast cancer is almost always due to gene amplification, HER2 amplification status can be assessed by ISH. In the case of TNBC, there is neither HER2 gene amplification nor protein overexpression.
Because of the absence of ER/PR expression or HER2 gene amplification/protein over-expression, and because of TNBC’s general poor outcome, it is generically treated with chemotherapy. However, molecular data – largely based on transcriptional +/- gene copy number analyses – have further defined TNBC into several subgroups, which has led to the discovery of potentially clinically actionable therapeutic targets. Right now, there are many ongoing clinical trials of targeted therapies and immunotherapies for TNBC. It is likely that patients’ responses to these therapies will be determined by their specific TNBC subtype, which means that the pathology laboratory will need to perform TNBC subtyping. Unfortunately, molecular-based subtyping, which includes transcriptional and/or gene copy number analysis, is relatively difficult to access and expensive to perform.
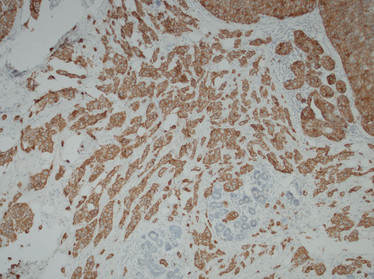
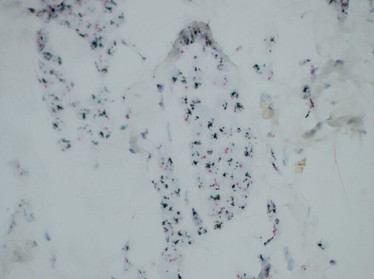
Figure 2. HER2-positive breast cancer. A) IHC shows strong overexpression (3+) of HER2 protein, a cell membrane protein. The invading cancer surrounds normal breast lobules, which do not overexpress the HER2 protein. B) ISH using a dual probe, with red dots hybridized to the centromere of chromosome 17 and black dots hybridized to the HER2 gene on chromosome 17. There are numerous black signals compared with red, indicating high-level amplification of the HER2 gene.
TNBC subtypes
Probably the most widely accepted subtyping of TNBC is based on transcriptomic analysis of TNBC (1). Initially, six subtypes of TNBC were proposed: basal-like 1 and 2 (BL1 and BL2), immunomodulatory (IM), mesenchymal (M), mesenchymal stem-like (MSL), and luminal androgen receptor (LAR). What distinguishes these subtypes?
- BL1 subtype cancers are characterized by altered transcription of genes involved in the cell cycle, in cell division, and in DNA damage response pathways.
- BL2 subtype cancers show alteration of growth factor signaling as well as glycolysis and gluconeogenesis.
- IM subtype cancers are transcriptionally enriched for immune cell signaling.
- M and MSL subtypes are enriched for genes involved in cell motility, epithelial to mesenchymal transition and cellular differentiation pathways.
- In addition, MSL show altered growth factor signaling and angiogenesis. Interestingly, these cancers are said to express low levels of cell proliferation genes and also to have a low level of expression of claudin genes (previously described by other groups and exhibiting a particularly poor prognosis).
Although six subgroups were first described, the same researchers then compressed these six subtypes into only four (2), declaring that the IM and MSL transcriptional profiles were probably due to the transcriptional profiles of tumor-infiltrating lymphocytes and stromal cells, respectively, rather than of the tumor cells.
Other groups have proposed TNBC subtyping based on both transcription and gene copy number data (3). RNA and DNA genomic profiling have also been used to identify and confirm four distinct TNBC subtypes (4): LAR, M, basal-like immunosuppressed (BLIS), and basal-like immune activated (BLIA). BLIA was shown to have the best and BLIS the worst prognosis of these four groups. Ultimately, Ahn and colleagues (5) summarized TNBC subtypes that had been described independently by different groups and determined that the combined data identified four molecularly distinct TNBC subtypes, each amenable to different targeted therapies:
- basal-like, amenable to platinum based therapies and PARP inhibitors
- mesenchymal, amenable to MET, FGFR, and mTOR inhibitors
- immune, amenable to immune checkpoint inhibitors; and
- luminal androgen, amenable to androgen blockade and PIK3CA inhibitors.
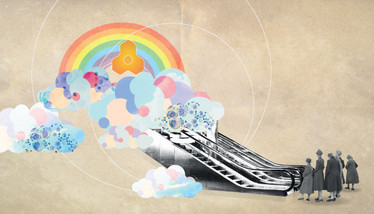
The future of TNBC
We are on the cusp of offering targeted therapies to TNBCs. However, current diagnostic categorization of breast cancers is based on ER/PR and HER2 expression, which is overly simplistic – and will be even more so when targeted therapies for TNBC subtypes are available. We will need a robust mechanism of identifying the different TNBC subtypes.
A corollary of developing targeted therapies for TNBC subtypes is to redefine our interpretation of what exactly is “triple-negative.” Prior to any targeted therapies, if even a low level of ER expression was detected in a breast cancer, it would be treated as an ER-positive breast cancer and the patient offered hormone therapy. However, ample data suggest that some low-ER-expressing breast cancers behave, in both outcome and response to hormone therapies, much more like TNBC (Figure 3). Now and in the future, though, categorizing such cancers as ER-positive – rather than as one of the TNBC subtypes –would likely mean denying that patient much more effective TNBC-targeted therapy. Therefore, not only will we have to diagnose different TNBC subtypes, but we will also have to positively identify cancers that would be amenable to TNBC targeted therapies, despite some (usually weak and focal) expression of ER/PR.
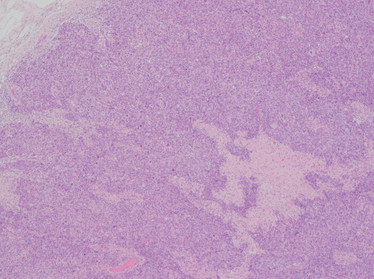
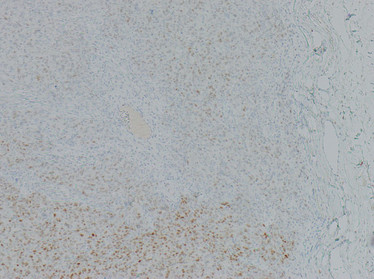
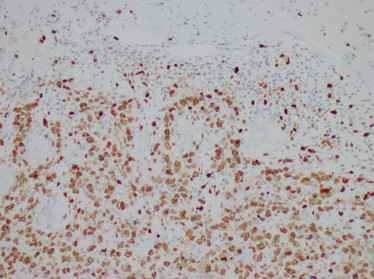
Figure 3. This breast cancer has the classical morphology of basal-like breast cancer (i.e., TNBC), but would be classified as ER-positive (non-TNBC) due to weak-to-moderate focal ER expression detected by IHC. A) This high-grade breast cancer has morphological features of a basal-like breast cancer (H&E stain). B) The same cancer shows focal ER expression and is therefore classified as an ER-positive breast cancer. C) The cancer has a very high Ki-67 expression index (>90 percent of tumor cells positive), indicating a high proliferation rate – another feature of basal-like breast cancers.
My personal belief is that even small-panel genomic analysis of breast cancer is too expensive to be used in a diagnostic sense to identify TNBC subtypes. I also suspect that there is probably insufficient skilled manpower to interpret genomic data for such a common cancer – and, even then, any technique that does not identify and analyze tumor cells within a population of non-tumor cells in a tissue sample may lead to an erroneous result. Equally, given the advent of immune therapies, we are now interested in the interplay between the genomics of a tumor and the patient’s response to that tumor – in effect, a precision medicine approach to therapeutics.
The interplay between the tumor and the patient’s individual reaction is presented and captured in the microscopic morphology of that tumor. In fact, it is possible for a breast pathologist to diagnose basal-type and luminal androgen receptor-type TNBC on morphology supported by selective IHC. This morphology can be recognized even if the tumor also weakly expresses ER/PR. It will also disclose the extent of any immune response to the tumor that may be amenable to immune checkpoint inhibitors. Therefore, I believe that we should take a renewed interest in the morphological classification of breast cancers – and possible expansion of the morphological classification to identify new determinants of tumor outcome – by analyzing the patient’s individual response to the tumor, perhaps by accurate assessment of tumor infiltrating lymphocytes or stromal-tumor interface factors.
It is likely that histopathologists’ standard morphological analysis of breast cancer can be greatly enhanced by applying digital image capture, artificial intelligence (AI), and deep learning techniques to tumor morphology. Such an approach may consolidate the morphological factors identified within a tumor section that relate to that tumor’s molecular constitution, and hence its TNBC subtype. It may also be able to recognize new morphological factors, such as tumor-stromal interactions, that are important for tumor outcome.
We also know that digital image analysis of tumor sections is more accurate than pathologists’ interpretations of important quantitative data, such as the quantification of tumor infiltrating lymphocytes. And that’s why I think it is important to revisit tumor morphology in the post-molecular era using these new techniques, which are capable of capturing and analyzing large sets of data on a similar scale to genomic data – except that the inputs are morphological. The ultimate outcome may be that digital imaging, AI, and deep learning techniques will help us spot previously unrecognized prognostic and predictive factors, and that digital image interpretation may be well-suited to assess quantitative data within a microscopic section. We can apply the integration of these techniques, and the enhanced morphological analysis they will yield, to accurately interpret different types of TNBC.
My colleagues and I have developed an international collaboration to investigate TNBCs via digital image capture and AI on the background of known patient outcome, standard morphology assessment, and genomic data. We are performing this research to clarify how morphology can be used to subtype TNBC.
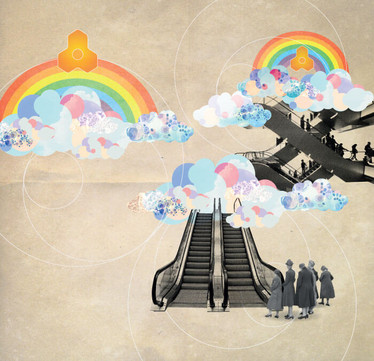
A part of the future
My own career as a histopathologist has spanned almost 30 years and definitely started in the pre-genomic era. I have seen the giant leap we have made in patient outcomes, partly by integrating molecular data into patient management. I am also interested in seeing just how far immune therapeutics can be successfully applied to cancer management. But throughout all these developments, I have held on to the belief that the histopathologist, with their detailed knowledge of disease processes and their visual knowledge of the complexity of disease, should be at the forefront of integrating new techniques into diagnosis.
I think that we have to be wary of “sound bite” pathology that is easy for non-pathologists to understand, such as the categorization of weak ER-expressing cancers as “ER-positive” when, in fact, the complex morphological information (much more difficult for a non-morphologist to comprehend) suggests that it should be in a different category of breast cancer. Therefore, my recommendation would be for an integrated approach to breast cancer diagnostics, where morphology is understood to be the visual picture of tumor–host interaction – and, therefore, the foundation of precision medicine.
- BD Lehmann et al., “Identification of human triple-negative breast cancer subtypes and preclinical models for selection of targeted therapies”, J Clin Invest, 121, 2750–2767 (2011). PMID: 21633166.
- BD Lehmann et al., “Refinement of triple-negative breast cancer molecular subtypes: implications for neoadjuvant chemotherapy selection”, PLoS One, 11, e0157368 (2016). PMID: 27310713.
- C Curtis et al., “The genomic and transcriptomic architecture of 2,000 breast tumours reveals novel subgroups”, Nature, 486, 346–352 (2012). PMID: 22522925.
- MD Burstein et al., “Comprehensive genomic analysis identifies novel subtypes and targets of triple-negative breast cancer”, Clin Cancer Res, 21, 1688–1698 (2015). PMID: 25208879.
- SG Ahn et al., “Molecular classification of triple-negative breast cancer”, J Breast Cancer, 19, 223–230 (2016). PMID: 27721871.
Clinical Director, Tissue Pathology and Diagnostic Oncology, ICPMR, Westmead Hospital at NSW Health Pathology and Clinical Professor of Pathology, Sydney University Medical School, Australia.