Unlocking the Value of RNA
RNA biomarkers lag behind DNA and proteins in clinical use because of the limitations of lab technology – but new analytical tools could now make RNA a useful tool in the pathologist’s kit
Our understanding of RNA is constantly evolving, with exciting discoveries like alternative splicing and RNA catalytic activity only a few decades old (1). The discovery of noncoding RNAs has opened up a new world of nucleic acid function, helping us to understand how life’s incredible phenotypic diversity arises from a relatively small, fixed set of genes. Amazingly intricate and dynamic, alternative splicing has the potential to produce any number of splice variants for a given gene. In fact, according to the most recently published human genome (2), we now have approximately 60,000 human genes transcribing into nearly 200,000 RNA species (see Figure 1 for a more detailed breakdown). The expression levels of those RNAs are highly dynamic, integrating both genetic and epigenetic mechanisms of gene regulation to reflect the state of a biological system – which makes them an excellent choice as biomarkers.
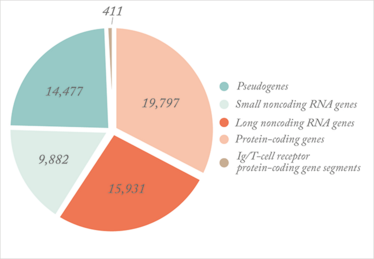
Figure 1. Current understanding of the division of human genes between protein-coding, RNA, immunoglobulin/T-cell receptor gene segments and pseudogenes.
Biomarkers, indicators of biological and disease states, have long been used for diagnostic testing – and in this era of personalized medicine, their importance is growing exponentially. All three of the main macromolecules in the cell – DNA, RNA and protein – can serve as valuable markers of a specific biological trait or measurable change directly associated with a physiological condition or disease status (3). But measuring the biomarkers themselves can prove challenging, as not all of the tools for doing so are created equal in terms of information obtained, technical advances or clinical compatibility.
Which biomarker is best?
Most biomarkers today are discovered as RNA, thanks to transcriptomic discovery programs that rely on the high-throughput capabilities of microarrays and RNA sequencing. Because RNA profiling benefits from rapid and relatively inexpensive next-generation sequencing (NGS) and whole-genome microarrays, RNA is an ideal biomarker choice – and yet, it remains difficult to translate discoveries to the clinic for routine measurement. Why? The blame lies with established analytical technologies. Although it’s commonplace to detect and visualize native DNA and proteins in a single cell, the best routine measurement tools for RNA analysis detect and quantify it in solution, which results in a loss of morphological context. Because we lack appropriate methodology for measuring RNA in situ, many laboratories use DNA and protein as surrogates, which can be problematic for a variety of reasons (see Table 1).
Biomarker | Pros | Cons |
RNA | • Integrates genetic and epigenetic mechanisms of gene regulation • In situ measurement techniques are becoming more accessible • Many biomarkers are discovered as RNA from transcriptomic studies • Expression reflects the state of a biological system • Can detect biomarkers for which no antibodies are available | • Compared to DNA and protein, RNA is more susceptible to degradation during specimen handling and storage, demanding more robust method of detection • RNA work requires special care in the laboratory to ensure an environment free of ubiquitous ribonucleases |
DNA | • Single-copy in situ detection is possible through FISH • Can detect structural alterations (translocations, deletions, amplifications) • Specificity is easy to verify visually • NGS offers many benefits | • DNA alterations don’t always lead to RNA or protein expression changes • Conventional FISH can’t detect DNA alterations at single-gene resolution and is unsuitable for detecting small DNA alterations (microdeletions, micro-amplifications, gene rearrangements) • NGS requires sophisticated bioinformatics and offers no morphological context |
Protein | • Functional components of a cell • Well-established methodologies are available, rapid and easy to perform • In situ detection for routine use is available • Options for automation exist • Can be cost-effective when antibodies are available | • Limited antibody availability and quality • Development and validation of new antibodies can be slow and expensive • Limited detection of secreted and scarce proteins • Only works on protein- coding genes • Unsuitable as a surrogate for RNA |
The main issue with analyzing a protein in place of an RNA biomarker is that, because of gene regulation at the transcriptional and post-transcriptional levels, protein and RNA levels rarely exhibit a linear correlation. So even though the expression pattern of an RNA molecule may indicate a particular biological state, its protein counterpart may signal something very different. And for noncoding RNA molecules, which have no protein counterparts, measuring RNA is the only option. An additional complication is that most protein detection techniques – such as immunohistochemistry (IHC), the main method of protein biomarker detection in situ – rely on the use of antibodies, whose quality can be limiting or prevent the detection of scarce or secreted proteins. In some cases, no antibody to the protein of interest exists at all, meaning that researchers must either develop and validate their own over time, or find an alternative detection method.
Like RNA and proteins, DNA biomarkers are also important in research and clinical applications. This is especially true because many structural alterations to DNA are linked to cancer and other diseases, so it’s important to look at the DNA itself using techniques like DNA fluorescence in situ hybridization (FISH), polymerase chain reaction (PCR) and evolving NGS technologies. But structural rearrangements don’t always result in changes to transcriptional activity or levels of expression – so an increased DNA copy number does not necessarily translate to an increased amount of RNA or protein (4).
As DNA and protein surrogates may not recapitulate the information provided by an RNA biomarker, and solution-based RNA analysis takes the expression out of its cellular context, what’s the best way for laboratory medicine professionals to perform routine biomarker profiling following transcriptomic exploration? The answer lies in direct RNA measurement in situ. And at the moment, there’s an unprecedented demand for effective tools to do just that. To gain biological insights, we need the ability to not only detect the RNA, but also to localize it in the cell. It’s this combination of detection and localization that researchers are targeting now in order to take advantage of RNA’s potential as an indicator of health and disease.
The key to precise localization
First applied to RNA in 1981 (5,6), in situ hybridization (ISH) can effectively determine the precise localization of target RNA in single cells. The technique provides the spatial and morphological context we need to understand the physiological and pathological relevance of a given target RNA. For instance, we can detect RNA expression in specific cell types to distinguish between stromal and tumor expression (see Figure 2), or detect it in rare cell populations such as cancer stem cells or circulating tumor cells. So if the answer to the RNA measurement conundrum lies in a technique that was introduced decades ago, why is it not widely used? In the past, ISH was not only time-consuming and difficult to use, but it lacked sensitivity and specificity – deficits that presented a barrier to its becoming commonplace in research and diagnostic pathology labs. But now that there have been some significant advancements to ISH techniques, the outlook is changing.
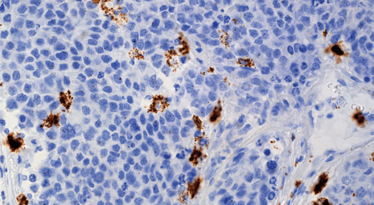
Figure 2. Human breast cancer tissue probed for expression of the MMP9 mRNA, which codes for a matrix metalloprotease whose overexpression facilitates tumor growth and invasion. The signal (brown) is abundant in scattered stromal cells of the tumor microenvironment, but is rarely expressed within the tumor cells themselves (12).
Nowadays, we have multiplex nucleic acid in situ hybridization technologies that overcome those limitations, enabling single-cell gene expression profiling in situ with detection sensitivity and specificity down to a single molecule (see Figure 3). Incorporating a variety of innovative approaches to probe design and signal amplification, these recent advances (7–11) take in situ RNA detection to an entirely new level. Single-molecule detection allows single-cell transcript quantification to be as simple as counting dots in a cell, which can be automated with advanced image analysis software. Robust probe design strategies also provide compatibility with partially degraded RNA, allowing for robust RNA detection in routine clinical specimens like FFPE tissue. Adopting these techniques on commonly used automated slide staining instruments minimizes manual labor and variability, facilitating seamless integration of RNA biomarker assays into existing pathology lab workflows. The time has come to unlock the full potential of RNA biomarkers.
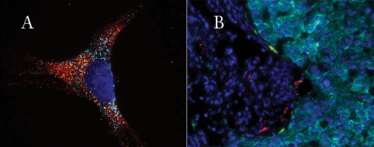
Figure 3. A. HeLa cell hybridized with probes to β-actin, hypoxanthine phosphoribosyltransferase 1 (HPRT-1), 60S acidic ribosomal protein P0 (RPLP0), and peptidylprolylisomerase B (PPIB) in multiplex fluorescence format. The nucleus is counterstained with DAPI. B. Multiplex fluorescence detection of uPA and PAI mRNAs in breast cancer. Merged pseudo-colored image of a metastatic breast cancer tissue section stained with probes specific to cytokeratins [PanCK (CK-8, CK-18, and CK-19), aqua], uPA (red), and PAI-1 (green) (11).
Because it’s central to the flow of genetic information in the cell, RNA is ideally positioned to reflect cellular physiology, making it an ideal biomarker. The limitations of DNA and protein surrogates, and of analyzing RNA in solution, make it clear that there is no substitute for measuring RNA itself in situ – and thereby providing a direct path from the lab to the clinic. While NGS approaches continue to fuel RNA biomarker discoveries, quantitative RNA biomarker analysis that includes tissue morphology at single-cell resolution, will facilitate rapid validation of novel RNA biomarkers and help us translate them into clinical use.
- M Guttman, et al., “Chromatin signature reveals over a thousand highly conserved large noncoding RNAs in mammals”, Nature, 458, 223–227 (2009). PMID: 19182780.
- Wellcome Trust Sanger Institute, “Statistics about the current Human GENCODE Release (version 23)” (2015). Available at: bit.ly/1VACUwH. Accessed July 23, 2015.
- MW Pfaffl, “Transcriptional biomarkers”, Methods, 59, 1–2 (2013). PMID: 23312615.
- MW Wynes, et al., “FGFR1 mRNA and protein expression, not gene copy number, predict FGFR TKI sensitivity across all lung cancer histologies”, Clin Cancer Res, 20, 3299–3309 (2014). PMID: 24771645.
- HA John, et al., “RNA-DNA hybrids at the cytological level”, Nature, 223, 582–587 (1969). PMID: 5799530.
- LM Angerer, RC Angerer, “Detection of poly A+ RNA in sea urchin eggs and embryos by quantitative in situ hybridization”, e, 9, 2819–2840 (1981). PMID: 6169002.
- A Raj, et al., “Imaging individual mRNA molecules using multiple singly labeled probes”, Nat Methods, 5, 877–879 (2008). PMID: 18806792.
- HMT Choi, et al., “Programmable in situ amplification for multiplexed imaging of mRNA expression”, Nat Biotechnol, 28, 1208–1212 (2010). PMID: 21037591.
- C Larsson, et al., “In situ detection and genotyping of individual mRNA molecules”, Nat Methods, 7, 395–397 (2010). PMID: 20383134.
- KH Chen, et al., “Spatially resolved, highly multiplexed RNA profiling in single cells”, Science, 348, aaa6090 (2015). PMID: 25858977.
- F Wang, et al., “RNAscope: a novel in situ RNA analysis platform for formalin-fixed, paraffin-embedded tissues”, J Mol Diagn, 14, 22–29 (2012). PMID: 22166544.
- H Wang, et al., “Quantitative ultrasensitive bright-field RNA in situ hybridization with RNAscope”, Methods Mol Biol, 1211, 201–212 (2014). PMID: 25218387.
Xiao-Jun Ma is chief scientific officer at Advanced Cell Diagnostics, Hayward, CA, USA.