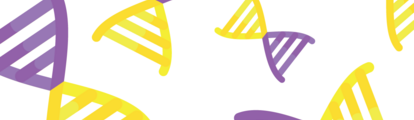
Testing for Gene Fusions
A comparison exercise among commonly used NGS assays
sponsored by Thermo Fisher Scientific
Based on an educational webinar with Wei Song and Phillip Jermann
Genetic rearrangements with contiguous, but unrelated, nucleic acid sequences – so-called fusion genes – often drive malignant transformation (1). Their identification is important and growing in precision oncology research, but can present some challenges. First, it can be time-consuming. Second, the trend toward smaller biopsies and limited tumor content makes high demands on assay performance, including starting input and limit of detection (LoD). Finally, the continuous discovery of new oncogenic rearrangements means that specific assays are not available for all fusion genes.
Can recent advances in NGS instrumentation and solutions address these difficulties?
NGS-based tests that use RNA (rather than DNA) as the input analyte are particularly useful for fusion detection. These RNASeq assays enable us to both detect the fusion and also to measure its expression level. Furthermore, unlike DNA-based tests, RNASeq assays can accommodate sequences with large intronic regions (for example, NTRK genes). But how do these tests perform in the real world? After all, for a gene fusion assay to be useful, it must meet specific requirements – not least the following:
- Rapid turnaround time to permit simultaneous return of both NGS and immunohistochemistry data (ideally in a fully integrated report)
- Minimal input requirement to consistently accommodate very small biopsies and low tumor cell counts; the ability to detect gene fusions from low transcript levels and retain high sensitivity and specificity without an unacceptable level of false positives
- Reliably identify all known gene fusions, as well as novel fusion isoforms
How do existing RNASeq technologies perform against the above criteria
The Song laboratory has compared the ability of three different NGS-based RNASeq tests – the FusionPlex™ Solid Tumor (FPST) from ArcherDx, the TruSight™ Oncology 500 (TSO500), and the Oncomine™ Comprehensive Assay v3 (OCPv3) – to detect NTRK fusions. First, Song’s team showed that all three assays successfully detected the fusions present in the SeraSeq RNA standards for which they had compatible probes (FPST and TSO500 detected 15/15 SeraSeq FFPE NTRK fusion samples, and OCPv3 detected 13/13). Next, they assayed 16 clinical research specimens from glioblastoma patients, all bearing either an NTRK1 or NTRK3 fusions, except one with an NTRK2 fusion. Although the TSO500 detected all fusions, FPST and OCPv3 did not detect the NTRK2 rearrangement. Finally, again using the SeraSeq RNA standards, Song’s lab compared the assays’ LoDs using a dilution-based approach.
Notably, LoD varied substantially between platforms; for example, to detect the TPM3-NTRK1 fusion, the TSO500 required ~20 copies, whereas FPST and OCPv3 required only one copy; for ETV6-NTRK3, the FPST and TS500 required ~50 copies and the OCPv3 needed only one copy; and lastly, for LMNA-NTRK1, the TSO 500 needed ~20 copies, the FPST two copies, and OCPv3 only one copy. Finally, although these examples highlight that LoD is a major differentiator between the three assays, another critical parameter enhancing the differences between them is the assay input requirements. The FPST requires 25–250 ng input RNA, TSO500 requires 45–85 ng, and OCPv3 needs only 1–20 ng.
Jermann’s team evaluated the recently launched Oncomine™ Precision Assay, in combination with the latest Ion Torrent™ sequencer, the Genexus™ System. This new panel, unlike the OCPv3 assessed by Song’s team, uses novel FusionSync™ technology to identify both known and novel gene fusions (see sidebar: Detecting known and novel fusions with FusionSync). By testing SeraSeq RNA standards, Jermann showed that the Oncomine™ Precision Assay detected all known driver gene fusions and NTRK rearrangements. The subsequent analysis of clinical research samples showed 100 percent detection concordance (see Figure 1) with reference methods (FISH or FPST assays) used for specimen characterization, along with demonstrating the added value of both methodological elements constituting the FusionSync™ technology – the targeted part and the tiling imbalance – with respect to their ability to identify both the driver gene and the break point of the rearrangement.
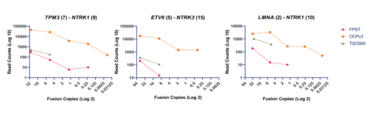
Figure 1. LoD comparison. OCPv3 enables the detection of low abundant fusion events, requiring lower fusion copies to generate higher read counts compared with FPST or TSO500.
Could this be the future of fusion detection?
Song’s evaluation shows that OCPv3 has the best-performing LoD of the three tested assays, along with lower input requirements. Nonetheless, the OCPv3 could not detect unknown novel fusions, representing a drawback for investigating tumor types in which the landscape of gene fusions has not yet been thoroughly investigated. This limitation does not apply to FPST or TS500. Conversely, Jermann’s experiments show that the new Oncomine™ Precision Assay on the Genexus™ System overcomes this limitation – without compromising the typical Oncomine™ characteristics. The Oncomine™ Precision Assay requires only 10 ng of input nucleic acid, which helps laboratories achieve minimal sample rejection rates while working with increasingly small biopsies.
The unprecedented level of automation in the Genexus™ System has a direct impact on time to results; the 5–10 days usually required for manual NGS systems can now be reduced to 1–2 days. Such a substantial reduction of the workflow turnaround time enables laboratories to provide both NGS and immunohistochemistry results at the same time as part of a single integrated report. Lastly, but not least, the fully automated NGS technology embodied in the Genexus™ System considerably lowers a major NGS uptake barrier by removing the need to employ highly specialized NGS technicians exclusively for NGS-related operations, extending the audience of laboratories that could embrace this technology.
Wei Song, Director of the Clinical Genomics Laboratory at Weill Cornell Medical College, works on novel methods for analyzing the mutation profiles of cancers, with a particular focus on application of NGS to define the mutation profiles of solid tumors.
Phillip Jermann, Head of Molecular Assay Development at the Institute of Medical Genetics and Pathology, University Hospital, Basel, has helped establish NGS-based diagnostic laboratories throughout Europe and works on development and evaluation of novel molecular diagnostic assays.
Detecting known and novel fusions with FusionSync™
FusionSync™ consists of two underlying technologies: i. Detection of known fusion isoforms (as per previous Oncomine™ assays) by reverse transcription of sample RNA into cDNA, followed by multiplexed PCR amplification of specific fusion genes; ii. Detection of fusions involving known driver genes (ALK, RET, FGFR1, 2 and 3 and NTRK1, 2 and 3) and unknown partners, by means of the tiling imbalance assay; such fusion events would have been missed by previous versions of Oncomine assays. The tiling assay detects expression imbalances between the 5' and 3' ends of the gene; such imbalances indicate that part of the gene has been translocated and is being controlled by a different promoter, as part of a chimeric gene product. The assay generates an imbalance score and a T value for deviation of expression from baseline. The resulting values are fed into an algorithm, which then returns an estimate of the probability of rearrangement.
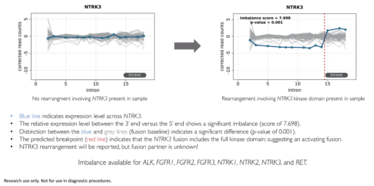
Figure 2. NTRK3 fusion analysis by FusionSync™. Exon expression levels in the test sample (blue line) are compared with baseline expression of non-rearranged DNA (grey lines; several readings are taken to account for sample-to-sample variation). A flat line (left) indicates absence of gene fusion. An expression imbalance (right) indicates rearrangement; here, the expression is below baseline until exon 15, and jumps above baseline thereafter. Red dotted line = predicted breakpoint.
- Q Gao et al., “Driver fusions and their implications in the development and treatment of human cancers”, Cell Rep, 23, 227 (2018). PMID: 29617662.