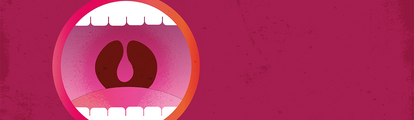
Breathe In, Breathe Out
Early detection of infectious disease is crucial for ensuring timely treatment and preventing further spread – could the answer lie in our breath?
Madeleine Ball | | 5 min read | Discussion
Accurately diagnosing infectious diseases in the clinic ensures appropriate treatment and increases our understanding of how an infection is moving through a population. Early detection is always welcome, but it is especially critical when it comes to rapidly progressing infections. Early detection also helps inform public health strategies to control transmission – a situation we became all too familiar with during COVID-19, where lateral flow tests enabled rapid detection of infection. That second pink or red line on the test drove behavior changes (social distancing and isolation at home) with the ultimate hope of controlling the spread of SARS-CoV-2. But to reliably inform timely treatment and optimal public health strategies, tests must be accurate, accessible, and easy to use.
The two most commonly used COVID-19 tests – PCR and at-home lateral flow tests – relied on nasal or nasopharyngeal swabs (and sometimes saliva) as sampling mediums. But other sampling mediums are also used in infectious disease diagnostic tests; for example, fecal sampling is used to diagnose food-borne illnesses caused by bacterial genera like Salmonella (1), and blood tests are used for bacteria, such as Staphylococcus aureus and Escherichia coli, and viruses, such as HIV and Ebola (2,3).
What about exhaled breath? An arguably underused sample for infectious disease diagnosis, breath is a virtually inexhaustible resource that is produced constantly by the body. A very large volume can be collected quickly and easily and, with certain methods, the volatile compounds in breath can be concentrated for higher sensitivity. All invasive sampling methods come with some sort of risk of infection or damage to the surrounding tissue, but breath collection is completely non-invasive, thereby removing these associated risks. Invasive tests are also generally more expensive than breath collection – an important consideration in the development of public health policies that must take budgeting and different priorities into account. This is especially relevant when considering the feasibility of implementing policies globally in resource-limited areas.
Exhaled breath is a complex and diverse matrix enriched with volatile metabolites – sometimes called volatile organic compounds (VOCs) – originating from both the physiological processes ongoing in the body and through inhalation from the environment. Infection exerts unique pressures on the body, causing host immune responses that can produce a different pattern of VOCs detectable in the breath. Microbial pathogens can also have their own metabolic processes – the metabolites of which can be identified in the breath.
One example currently in clinical practice is the breath test for detecting a Helicobacter pylori infection of the stomach. Residing in approximately 50 percent of the global population’s stomach (4), H. pylori is the causative agent of chronic gastritis. Importantly, its presence is the strongest known risk factor for developing gastric cancer (5, 6). H. pylori can be detected by ingesting a substrate solution containing 13 Carbon Urea (13C-Urea), and subsequent collection of a breath sample 30 minutes afterward. Unlike other cells in the stomach, H. pylori can metabolize urea into ammonia and carbon dioxide; therefore, the presence of an H. pylori infection is indicated by elevated levels of these compounds in the breath. Many more characteristic changes in breath composition could be detected and associated with specific infections, potentially even before symptoms have manifested.
Changes in the levels of volatile metabolites in exhaled breath following infection have so far been identified for influenza A and B, parainfluenza 1, 2, and 3, respiratory syncytial virus, human metapneumovirus, human rhinoviruses, tuberculosis, aspergillosis, and many more (7, 8, 9, 10, 11). In other words, clear evidence lies behind the rationale that the presence of microbes leads to characteristic changes in the VOCs in breath, which could be used to develop diagnostic tests for infectious disease.
Breath analysis was a promising option during the pandemic for rapidly diagnosing COVID-19. In 2022, the FDA issued emergency authorization of the InspectIR COVID-19 Breathalyzer that could identify compounds in the breath associated with SARS-CoV-2 in less than three minutes (12). This test used gas chromatography-mass spectrometry (GC-MS) analysis – a technique that separates volatile compounds in breath – and produces a dataset of mass spectra consisting of a series of peaks that vary in size and shape depending on the abundance and chemical identity of the compounds. Similar to at-home PCR sampling for COVID-19, there is no requirement for patients to visit a clinic to take breath tests because breath can be collected anywhere and sent to a lab for analysis.
Portable sensor-based breath testing devices have also been developed that detect the pattern – or “breathprint” – of exhaled breath, rather than identifying individual compounds. However, identifying each volatile compound can help associate potential biomarkers in breath to the underlying biology, and sensor-based techniques can suffer from a lack of repeatability, as well as technical issues such as drift and sensor faults (13, 14). GC-MS is therefore considered the gold standard for analyzing VOCs within exhaled breath because of its sensitivity and ability to both identify and quantify specific compounds.
There are many benefits of using the breath as a sampling medium, making it an excellent method for diagnosing infectious disease. It is easy to collect, and GC-MS offers an accurate and accessible platform for analysis. The non-invasiveness of breath collection is especially appealing to patients and clinicians and is an important factor for increasing the uptake of such tools.
Although further research is needed into the characteristic changes of breath composition in response to infection, it is clear that implementing breath tests for a wider range of communicable diseases could revolutionize monitoring and diagnostics.
This article was originally published on our sister-brand, ID:Transmission.
- AC Chirambo et al., “Performance of molecular methods for the detection of Salmonella in human stool specimens,” Wellcome Open Res, 5, 237 (2021). PMID: 34017923.
- ML Wilson, “Development of new methods for detecting bloodstream pathogens,” Clin Microbiol Infect, 26, 319 (2020). PMID: 31425742.
- J Louten, “Detection and Diagnosis of Viral Infections,” Essential Human Virology, 111. Academic Press: 2016.
- M Zamani et al., “Systematic review with meta-analysis: the worldwide prevalence of Helicobacter pylori infection,” Aliment Pharmacol Ther, 47, 868 (2018). PMID: 29430669.
- JR Warren, B Marshall, “Unidentified curved bacilli on gastric epithelium in active chronic gastritis,” Lancet, 1, 1273 (1983). PMID: 6134060.
- P Correa, “Human gastric carcinogenesis: a multistep and multifactorial process--First American Cancer Society Award Lecture on Cancer Epidemiology and Prevention,” Cancer Res, 52, 6735 (1992). PMID: 1458460.
- S Traxler et al., “VOC breath profile in spontaneously breathing awake swine during Influenza A infection,” Sci Rep, 8, 14857 (2018). PMID: 30291257.
- J Gralton et al., “Respiratory virus RNA is detectable in airborne and droplet particles,” J Med Virol, 85, 2151 (2013). PMID: 23959825.
- AMI Saktiawati et al., “Diagnosis of tuberculosis through breath test: A systematic review,” EBioMedicine, 46, 202 (2019). PMID: 31401197.
- S Koo et al., “A breath fungal secondary metabolite signature to diagnose invasive aspergillosis,” Clin Infect Dis, 59, 1733 (2014). PMID: 25342502.
- CC Kennedy, RR Razonable, “Fungal Infections After Lung Transplantation,” Clin Chest Med, 38, 511 (2017). PMID: 28797492.
- FDA, “Coronavirus (COVID-19) Update: FDA Authorizes First COVID-19 Diagnostic Test Using Breath Samples” (2022). Available at: https://bit.ly/3E4e9FT.
- P Haripriya, “Breath VOC analysis and machine learning approaches for disease screening: a review,” J Breath Res, 17 (2023). PMID: 36634360.
- P Le Maout et al., “Polyaniline nanocomposites based sensor array for breath ammonia analysis. Portable e-nose approach to non-invasive diagnosis of chronic kidney disease,” Sens Actuators B: Chem, 274, 616 (2018).
Scientific Content Writer at Owlstone Medical, Cambridge, UK.