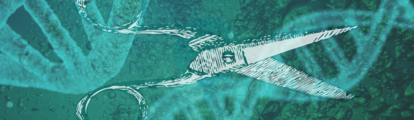
A Clean (Gene) Break
CRISPR/Cas gene editing is a powerful, ever-evolving tool – but it seems to be here to stay…
At a Glance
- Genome engineering technology has existed for a long time, but our newest tool, CRISPR/Cas, is our fastest and most efficient yet
- The technique still needs refinement; some attempts work well and others not at all – but we don’t understand why
- Solving those mysteries could give us a powerful tool for gene therapy and diagnostics
- CRISPR/Cas is so promising that I expect it go the way of PCR, becoming ubiquitous in all laboratories and – one day – clinics as well
Precise genome engineering isn’t new – neither the idea of it, nor its execution. The concept of genome engineering has been around for a long time. Humans have always thought that it would be fanciful if you could go into the inner workings of an organism to make it do – or even be – something different. The ability to actually do that in lower organisms has also been around for quite a long time; in fact, the reason yeast is so commonly used as an experimental model system is because of a 1980s research breakthrough when we figured out how to replace its genes. Essentially, technology was developed to change the yeast genome to anything we wanted! Importantly, during the course of that work, we also learned that if you created a double-stranded break in the chromosome, it greatly augmented the gene editing process – a seminal observation that reverberates all the way through to our newest and best technology: CRISPR/Cas.
But after this success with yeast, researchers tried to do similar things with higher organisms and discovered that it didn’t work nearly as well in most other eukaryotic cells. So for many years, people just threw pieces of DNA into mammalian cell lines and developed esoteric selections, trying over and over again to achieve practical gene editing. They only started to see success when they began applying the same double-stranded break trick they’d used in yeast. And that is, at the end of the day, the critical contribution of CRISPR/Cas – that it allows you to make a break wherever you want in the human genome.
The quality trade-off
That’s the plus side. The minus side is that it’s not perfect; you have to worry about off-target effects when you’re introducing chromosomal breaks. In our case – and many others – speed and efficiency really win out, and in that realm, CRISPR/Cas is superior to any other technique. But there’s a dichotomy here between basic research and clinical applications. For basic research, we want the “quick and dirty” solution. We want to know the approximate answer as fast as possible, and then we’ll keep investigating that until we can confirm it.
Clinical application is the other side of the coin. And there, all of a sudden, you really need to put on the brakes. Even a single alteration that you don’t want when you’re putting cells back into a human patient could cause significant harm. So for that use, you need your gene editing to be as perfect as it can possibly be. We can afford some “sloppiness” in the system when we’re performing basic research, but when it comes to patient care, that is – of course – unacceptable.
Need to Know
What do potential CRISPR/Cas users need to think about before starting experiments?
- Know how well your cell line takes up DNA. If you’re working with a cell line that takes up DNA very well, you know that you’re likely to be able to get your reagents into your cells – obviously a necessary first step in the process. If you’re working with one of the more esoteric human cell lines that isn’t very receptive to DNA uptake, that’s an important thing to consider before you start.
- Know how quickly your cell line proliferates. CRISPR/Cas editing works best in proliferating cells – but certain tissues are largely made up of non-proliferating cells. In those tissues, the editing process doesn’t work very well. You need an S-phase DNA replication to push it along.
- Know the ploidy of your cell. Ploidy doesn’t matter as much with CRISPR/Cas as it does with other methods – but that doesn’t mean you don’t have to think about it. When we started gene editing, we always used diploid human cell lines, which are hard to get your hands on. Most human cell lines in culture have some sort of cancer background, and because of that, they tend to have more than 46 chromosomes each – which makes gene targeting very difficult. If you have four copies of the chromosome that carries your gene, you’ve got to modify all four of them. CRISPR/Cas is so much more efficient that aneuploidy isn’t a dealbreaker anymore – but it is still relevant to the process of trying to figure out which cell line you want to use and which gene you want to alter.
The Lamborghini of gene editing
The easiest metaphor to describe other methods’ relationship to CRISPR/Cas is that of a manual versus an electric typewriter, or a Toyota versus a Lamborghini. In principle, both of the former machines perform the same function: they allow you to type, or to drive from one place to another. But the latter are orders of magnitude better than the former. So CRISPR/Cas does the same thing as a TALEN or zinc finger (the best reagents that the field had previously utilized) – it just does it much, much better.
“Better,” in this case, is mostly in terms of speed. To make a CRISPR/Cas reagent takes 24 hours; to make a good TALEN or zinc finger can take months of work. In that space of time, you can do dozens of CRISPR/Cas experiments! In fact, the difference is even more extreme because CRISPR/Cas allows you to easily multiplex. In the old days, we had to go after one allele of one gene at a time, an incredibly laborious approach when making double or triple knockouts because we had to do each round of gene targeting independently. With CRISPR/Cas, you can edit multiple loci at precisely the same time, cutting your workload by months – sometimes even years.
Your mileage may vary
One thing we have found out about CRISPR/Cas is that not every attempt works equally well. In some cases, we design a guide RNA (the portion of CRISPR/Cas that tells the complex where to make the break) that should go to a particular locus in the human genome, throw our reagents in with it, and it cuts beautifully; we get very high-efficiency repair and recombination. But if we make another guide RNA – even one that’s fairly similar – we might find that it only kind of works. There’s a little bit of repair and recombination, but not much, and we don’t currently understand why that is. Annoyingly, we don’t know why the process doesn’t work with the same efficiency at all loci. I’ve had instances in my own laboratory where we’ve tried to engineer a locus and it just hasn’t worked. We usually get around that dilemma by moving our guide RNA up or down the chromosome just a little, and all of a sudden, we’ll hit paydirt. I assume it has something to do with the chromatin state at the locus of interest – one region is more heterochromatic than another, or the DNA is so crowded with transcription factors that the CRISPR/Cas complex can’t access it, or there are epigenetic modifications with which Cas isn’t compatible. We just don’t know; all we have right now is the empirical observation that CRISPR/Cas gene editing works better at some loci than at others. And additional technical issues – like cell lines that don’t take up DNA very well – don’t help matters.
So we have biological problems, and then we have technical problems layered on top of that. Between those two things, it seems almost impossible to predict how well CRISPR/Cas editing will work. You just have to do the experiment and see what happens. If it works really well, you jump up and down and then go on with your work; if it doesn’t, you try to optimize. Is the problem the fact that the reagents aren’t getting in? Is it that the enzymes aren’t cutting very well? Can you use a better ratio, or combination of, reagents? There are plenty of ways to skin that particular cat – but it can sometimes take quite a while.
The bench-to-bedside barrier
When you start to consider using CRISPR/Cas for therapeutic purposes, it’s somewhat of a different beast. We’re no longer talking about culturing incredibly resilient cells that are easy to access and difficult to damage. If you want to re-engineer a patient’s cirrhotic liver, there are all sorts of new issues. You’ve got to deliver the treatment to the liver without letting it reach other parts of the body; you’ve got to work in tissues made up of mature, non-proliferating cells; you’ve got to worry about efficacy and ensuring that enough cells are corrected to yield a therapeutic benefit. In some diseases, that might just require a 5–10 percent correction – but in others, you might have to modify most or all of the cells. And that’s something we’re not yet able to do.
Here, too, the issue of off-target effects rears its ugly head. In basic research, you can afford some sloppiness in your system; an extra double-stranded break here and there, for example, won’t affect your experiment – but it can certainly affect the survival of a human being. So once you start talking therapy, you also need to start talking about ensuring that the process is error-free. But does that mean there’s no hope for CRISPR/Cas in the clinic? I don’t think so! In fact, it’s already being used, and I expect that will only increase as we continue to develop the technique.
Reality check
Right now, I think there are two very clear applications. One is gene therapy. If you can get access to the tissues affected by a single-gene disorder, there’s no reason you can’t go in right now with CRISPR/Cas and change the “bad” DNA into “good” DNA. That’s especially true of immunological or ophthalmic disorders, where the tissue is easily accessible. I think we’re going to see enormous progress in the next 10 years or so as people start to attempt these kinds of gene editing treatments.
The other up-and-coming application is diagnostics. Human genome sequencing has essentially made it so that, within 20 years, every baby’s DNA will be sequenced at birth. Doctors will examine each genome and say, “Oops, it looks like this child is at risk for diabetes,” and offer preventative treatment. In order to do that, though, we’re going to have to gain a much better understanding of the genome. At the moment, we have a lot of what we call “variants of unknown significance” – polymorphisms whose effects we don’t yet understand. We can tell whether or not someone has a mutation at a particular locus, but we don’t necessarily know whether or not that mutation actually has a biological consequence. Fortunately, CRISPR/Cas will allow us to answer those questions very quickly. Because it’s so efficient, we’ll be able to make 100 different cell lines where we’ve changed 100 different nucleotides, and then test all of them to see how their functions have changed. That’s already ongoing, and in the very near future, it will allow us to figure out which pieces of DNA are biologically significant and which are not – and then, hopefully, fix those that are damaged.
Out of the “water bath” stage
It’s always hard to imagine what the future might bring. If you’d asked me three years ago if we’d ever have reagents much better than TALENs, I would have probably said no – but all of a sudden, along came CRISPR/Cas. Now, what’s up-and-coming is the field of CRISPR interference and CRISPR activation. Essentially, you inactivate the Cas part of the CRISPR/Cas complex so that it no longer makes a break, but still localizes to a specific portion of the human genome. Then you can modify the Cas to have totally new functions, like methylating nucleotides or moving nucleosomes. Why is that useful? Well, for instance, you can produce phenotypic outcomes without actually changing the DNA – just by modifying the epigenetics to allow expression or inactivation. That’s a very powerful tool, and it’s one we’re rapidly acquiring even now.
One of the scariest things about gene editing is just how fast the field is moving. The original Cas is already antiquated! It had a tendency to cut at low efficiency at extraneous sites, so laboratories have now developed more evolved versions with high-efficiency cleavage and reduced off-target effects – and that’s only in the past few years. So there’s no question that the system is here to stay. It’s like the polymerase chain reaction (PCR). PCR arrived one day and simply never left; it’s in constant use now. I see CRISPR/Cas the same way, and although there will be constant tweaks and improvements – just like PCR – I anticipate that, 20 or 30 years from now, everyone will still be talking about the technique and using it in their everyday work. It’s hard for me to imagine anything so revolutionary that it would supplant CRISPR/Cas.
In terms of the PCR comparison, I think we’ve progressed just beyond holding our tubes in different water baths. In the beginning, it seemed like, every six months, somebody was inventing new and different PCR techniques. It just kept getting better and more efficient. That’s where I think we are now with CRISPR/Cas – out of the water bath stage, but very much at the beginning of its evolution. We’ll keep on finding new and different applications for it, and eventually, it will become as much of a laboratory standby as tools like PCR are now.
Eric A. Hendrickson is Professor of Biochemistry, Molecular Biology and Physics in the University of Minnesota’s College of Biological Sciences, Minneapolis, USA.

While obtaining degrees in biology from the University of Alberta and biochemistry from Penn State College of Medicine, I worked as a freelance science and medical writer. I was able to hone my skills in research, presentation and scientific writing by assembling grants and journal articles, speaking at international conferences, and consulting on topics ranging from medical education to comic book science. As much as I’ve enjoyed designing new bacteria and plausible superheroes, though, I’m more pleased than ever to be at Texere, using my writing and editing skills to create great content for a professional audience.